Jeff Marshall (Cal Poly Pomona) andJessica Pilarczyk (University of Southern Mississippi)
“A Live Dragon” Beneath the Sea
In Māori culture, the Taniwha is a dragon-like beast that lives beneath the water, sometimes protecting seafarers, while at other times wreaking disaster on coastal communities (King, 2007). Māori lore tells of Taniwha that cause sudden upheavals and changes in the coastline, altering the shape of the land-ocean interface. In the wake of New Zealand’s 2016 Mw7.8 Kaikōura Earthquake, the Taniwha was evoked as a supernatural force behind coastal uplift, tsunami, and landslides (Morton, 2018). For New Zealand, the Hikurangi subduction margin is a formidable Taniwha, a “live dragon” lurking just offshore, ready to unleash powerful forces locked within its seismogenic zone. With multiple collaborative research efforts now underway, geoscientists are shedding light on the habits of this secretive dragon, revealing new understandings of the earthquake and tsunami hazards that threaten New Zealand’s coastline.
The SHIRE Project
The Hikurangi margin along the east coast of New Zealand’s North Island (Fig. 1) provides an optimal venue for investigating megathrust behavior and controls on seismogenesis (e.g., Wallace et al., 2009 and 2014). Along-strike variations in multiple subduction parameters, such as interface coupling, fluid flow, and seafloor roughness, can be linked to observed differences in megathrust slip behavior (seismic vs. aseismic), forearc mass flux (accretion vs. erosion), and upper-plate deformation (contraction vs. extension). Much of the forearc is subaerial and therefore ideal for geodetic and geologic studies, while the submarine areas are easily accessible for geophysical imaging and monitoring. The SHIRE Project, funded by the NSF Integrated Earth Systems (IES) Program, is a four-year, multi-disciplinary, amphibious research effort involving a team of investigators at five US institutions, as well as multiple international collaborators from New Zealand, Japan, and the United Kingdom. This project is designed to evaluate system-level controls on subduction thrust behavior by combining both on and offshore active-source seismic imaging, with onshore paleoseismic, geomorphic, and geodetic investigations. The project results will be meshed with existing geophysical and geological datasets, and analyzed through the lens of state-of-the-art numerical modelling. The overarching goal is to develop an integrated perspective of the physical mechanisms controlling subduction thrust behavior and convergent margin tectonic evolution. Importantly, this perspective will help to elucidate a clearer picture of megathrust earthquake and tsunami hazards along New Zealand’s Hikurangi margin.
The SHIRE Project has three principal components:
Geophysical imaging: Harm van Avendonk (UT Austin) and David Okaya (Univ. of Southern California) are leading the shoreline-crossing geophysical imaging investigations. Marine seismic multi-channel reflection data (MCS) and seismic refraction data recorded by ocean-bottom seismometers (OBSs) are being used to characterize the incoming Hikurangi Plateau, map the structure of the offshore accretionary prism, and document subducted sediment variations. Onshore recordings of offshore airgun shots, explosive shots, and local earthquakes will determine the structure of the upper plate and properties of the deeper plate boundary zone.
Paleoseismology and morphotectonics: Paleoseismic and geomorphic studies led by Jeff Marshall (Cal Poly Pomona) and Jessica Pilarczyk (Univ. of Southern Mississippi) will collect new field data to supplement ongoing coastal tectonics investigations conducted by collaborators at New Zealand’s GNS Science. This integrated data set will help resolve megathrust slip behavior over several seismic cycles, and constrain long-term coastal uplift and subsidence patterns. This component of the project includes a Research Experience for Undergraduates (REU) program, supervised by Marshall, that engages US students in collaborative New Zealand fieldwork.
Numerical Modelling: Demian Saffer (Penn State) and Laura Wallace (UT and GNS Science) will coordinate the integration and analysis of project data through numerical modeling conducted by a team of U.S. and international investigators. The geophysical and geological results will be combined with a range of existing data sets from other projects to constrain numerical models of the physical state of the interface and evolution of the margin over both long and short (seismic cycle) timescales. Model results will also quantify linkages between in situ conditions, fluid flow, behavior of the subduction thrust, and subduction margin development.
SHIRE Spotlight: Geomorphic & Paleoseismic Studies
The SHIRE Project’s onshore geomorphic and paleoseismic fieldwork is investigating seismic cycle deformation in the coastal fore arc, focusing on geologic records of land level changes produced by episodes of tectonic uplift and subsidence. Jeff Marshall, Jessica Pilarczyk, and their students are targeting field sites along the North Island east coast (Fig. 2) that compliment ongoing investigations by GNS collaborators Nicola Litchfield, Kate Clark, and Ursula Cochran (e.g., Litchfield et al., 2016; Clark et al., 2015; Cochran et al., 2006). During field seasons in 2017 and 2018, the two research teams conducted parallel studies, with Marshall focused on marine terrace records of coastal uplift, and Pilarczyk on marsh stratigraphic records of subsidence and tsunami.
Marshall and students (Fig. 3A-F) are mapping, surveying, and sampling uplifted paleo-shorelines and marine terraces to identify past earthquakes, and to evaluate net coastal uplift patterns. Their efforts focus on several key locations along the Hikurangi margin, including the Raukumaura Peninsula, southern Hawkes Bay, and central Wairarapa coast. Coseismic uplift events are preserved along much of the Hikurangi coastline as elevated paleo-shore platforms and abandoned beach ridges. Marine shells collected from uplifted platforms and overlying beach sediments provide radiocarbon age constraints on prehistoric earthquakes. In addition to localized fieldwork, the Cal Poly Pomona team is using recently acquired airborne LiDAR imagery (provided by GNS) to correlate uplifted paleo-shorelines between field sites (both from this project and prior studies). The LiDAR data incorporates detailed altitude information, which can be used to track lateral variations in terrace uplift along the coast. Marshall and students are also mapping and sampling flights of uplifted Pleistocene marine terraces along the coast to evaluate longer-term fore arc uplift rates and deformation patterns.
Terrace cover beds have been sampled for optically stimulated luminescence (OSL) geochronology, and for the identification of volcanic tephra and loess deposits of known ages. During the next two years, terrace mapping and sampling will be expanded to new areas and drone imagery will be recorded for structure-from-motion studies. Project students will conduct digital terrain analyses using regional topographic data to evaluate net deformation patterns, calculate morphometric indices, and outline morphotectonic domains. Overall, the efforts of the coastal uplift team will provide new constraints on the timing and spatial distribution of both short-term seismic cycle events, as well as longer-term cumulative deformation.
Pilarczyk and students (Fig. 3 G-I) are using coastal sediments to develop long-term records of Hikurangi earthquakes and tsunamis. Microfossils such as foraminifera are used to recognize both subtle and abrupt changes in sea level along a coastline. An abrupt change in sea level, caused by coseismic subsidence, indicates the occurrence of an earthquake and can be recognized along the coastline as a soil buried beneath subtidal sediments. Because certain microfossils have fidelity to the tidal frame, they can be used to assess how much a coastline subsided during an earthquake. They can also be used to identify tsunami deposits because they indicate transport of marine sediment into a coastal setting where such sediment does not occur naturally. In this way, radiocarbon dating and microfossil analysis on coastal sediments can be used to understand the timing and magnitude of past Hikurangi earthquakes and tsunamis. In 2017 and 2018, Pilarczyk and students embarked on a sediment coring campaign that targeted low-energy depositional centers (i.e., marshes, lagoons) along the Hawke’s Bay coastline. Their mission was to find evidence for past Hikurangi earthquakes that would supplement the short-term observational record by expanding the age range of known events to include centennial and millennial timescales. The team’s ongoing investigations have led to the identification of newly discovered events that will help to better understand the seismic hazard for coastlines facing the Hikurangi margin. ■
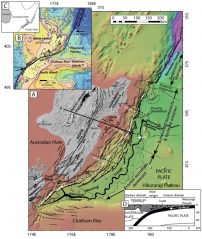
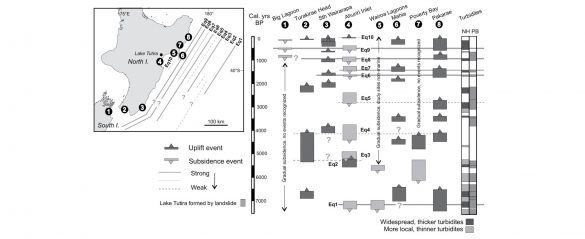